Introduction
Every year about 5,000–10,000 patients are diagnosed with esophageal diseases for which esophageal replacement is needed [Maghsoudlou et al., Tissue engineering of the esophagus, Seminars in Pediatric Surgery, (2014)]. Current choices for esophageal replacement involve the use of more distal parts of the gastrointestinal tract or colon, with long-term problems associated to these technique – such as graft failure, recurrent strictures, retarded voice rehabilitation and lifelong immunosuppression therapy. In view of such limitations, the immense potential offered by tissue engineering has been recognized and can be exploited as a good alternative for esophageal replacement. An esophageal scaffold must provide the necessary features – such as porosity, pore size and surface chemistry – to facilitate cell attachment and proliferation and the mechanical properties suitable to accomplish esophageal tissues function. Two biocompatible polymers have shown promising results for esophageal tissue engineering and for the creation of biocompatible and biodegradable patches for esophageal injuries: polylactic acid (PLA) and polycaprolactone (PCL). Scaffolds and patches can be prepared by solvent casting or electrospinning techniques.
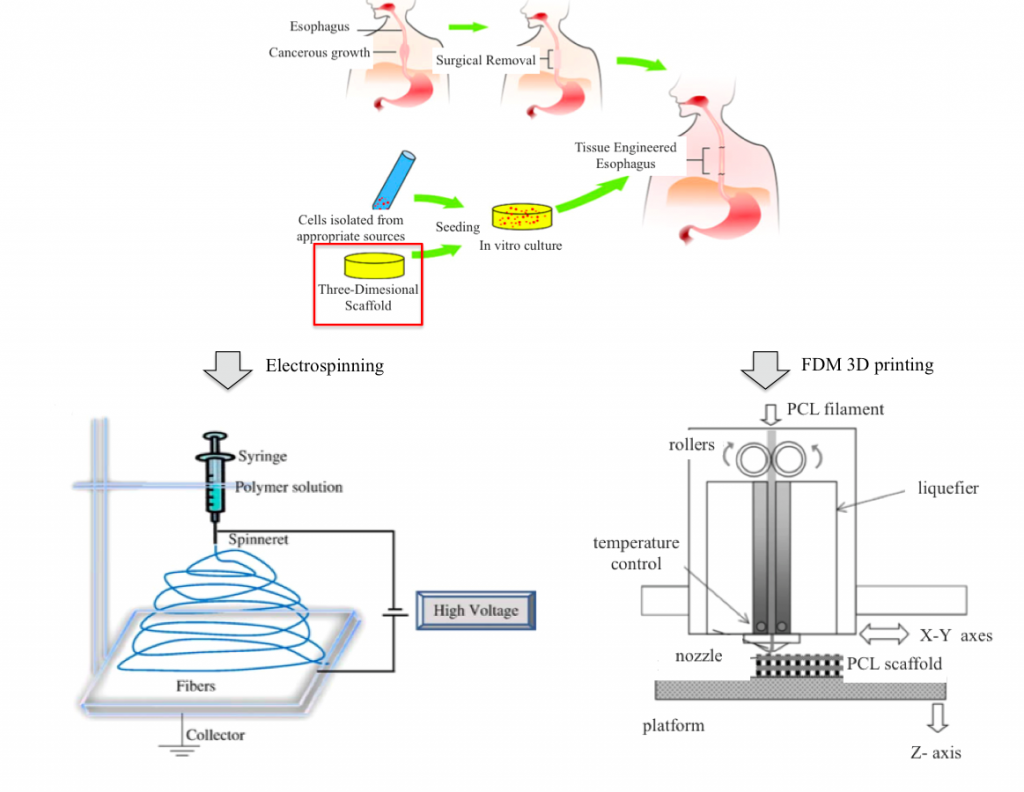
Fig. 1: Implementation procedure of a 3D scaffold using electrospinning or 3D printing
Idea
The project comes from the collaboration among the 3D printing laboratory ProtoLab of the Civil Engineering and Architecture Department (DICAr) and the Department of Pharmaceutical Sciences of the University of Pavia. The aim is to explore the potential of 3D printing technology on scaffold manufacturing in order to produce customized esophageal patches.
The idea is to print via FDM process co-polymeric PLA-PCL esophageal patches and to compare them with patches synthesized via solvent casting method in the Pharmaceutical Sciences lab. Solvent casting method is a powder blend technique for patches production through solubilization, freezing and lyophilization cycles.
3D printing allows to reproduce biological/geometric characteristics – such as dimensions and pores size – and mechanical properties of patches synthesized by our partners, drastically reducing production time and paving the way to innovative solutions tailored on the patient specific clinical case.
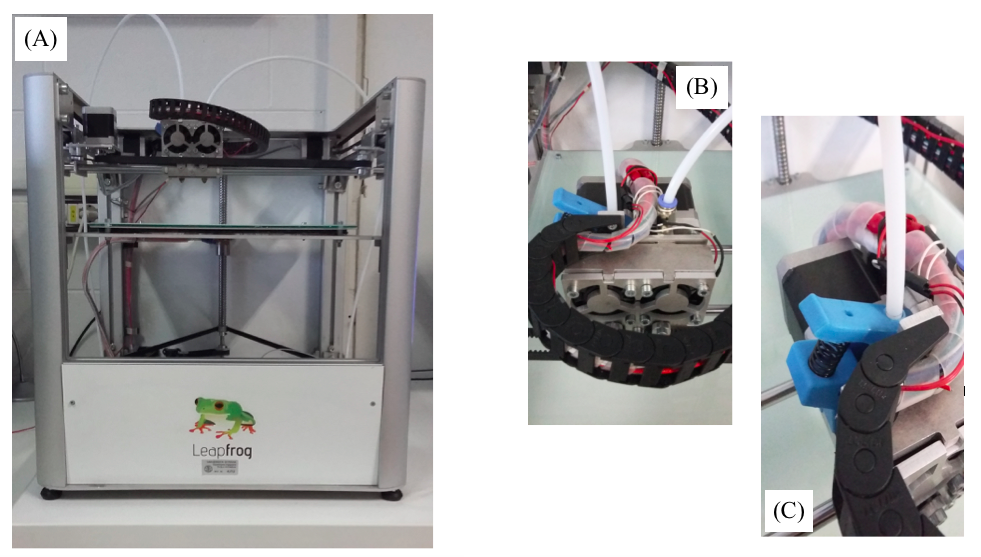
Both types of patches are subjected to biological characterization (cell adhesion and proliferation tests), mechanical characterization (tensile tests), physical-chemical and morphologic characterization (GPC, DSC, SEM) to compare the preparation techniques and their results. Conducted tests are:
- Gel Permeation Chromatography (GPC): determination of molecular weights of the used biopolymers before and after being processed.
- Differential Scanning Calorimetry (DSC): thermal analysis of polymers and copolymer before and after being processed.
- 3-(4,5-Dimethylthiazol-2-Yl)-2,5-Diphenyltetrazolium Bromide (MTT assay): cell attacchement and proliferation analysis.
- Scanning Electron Microscope (SEM): evaluation of pore size, shape and distribution throughout the patches.
- Tensile tests: mechanical characterization of patches.
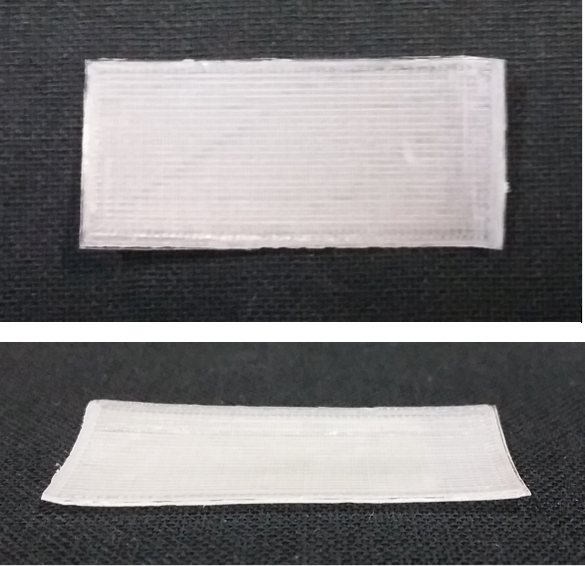
Fig. 2: PLA-PCL copolymer patch for esophageal tissue engineering 3D printed via FDM process
- Biological characterization of PCL and PLA thermoplastic filament
A sample of PCL filament and an extruded single PCL layer have been analysed by GPC in order to:
- Compare the PCL powder used in solvent casting method and the thermoplastic filament in terms of Mw, Mn and PI.
- Analyze how these parameters changes once extruded
- Setting of 3D printing parameters for PCL thermoplastic filament
Thermoplastic PLA filament is one of the most common materials used in the FDM process and its printing parameters are therefore already well-known. In our case, natural PLA filament requires a more complex set up due to the lack of any additives that can help the printing process. On the other side, the polymeric PCL wire is a completely new and uncharacterized material. It was then necessary to start setting the basic parameters – such as bed and extruders temperatures – and then refine the printing through more advanced options offered by Slic3r software.
- Setting of 3D printing parameters for a PLA-PCL double extruder printing
To print PLA-PCL copolymer it is necessary to use two extruders, one for each material. The set up for double extruder printing is a key aspect of this project and it is critical to the printing of copolymeric patches.
- Reproduction of biological and geometrical features of esophageal patches
To create patches comparable to the ones produced in the Drugs Science lab through solvent casting technique, we need to reproduce both their composition and structural characteristics. They must have analogue sizes, shape and pores structure. In a FDM process we can act on pores size parameter by changing the infill percentage. Specimens with different infill percentages were subjected to Scanning Electron Microscope (SEM) analysis in order to estimate the most suitable infill that generates a pores size comparable to the one obtained through solvent casting technique.
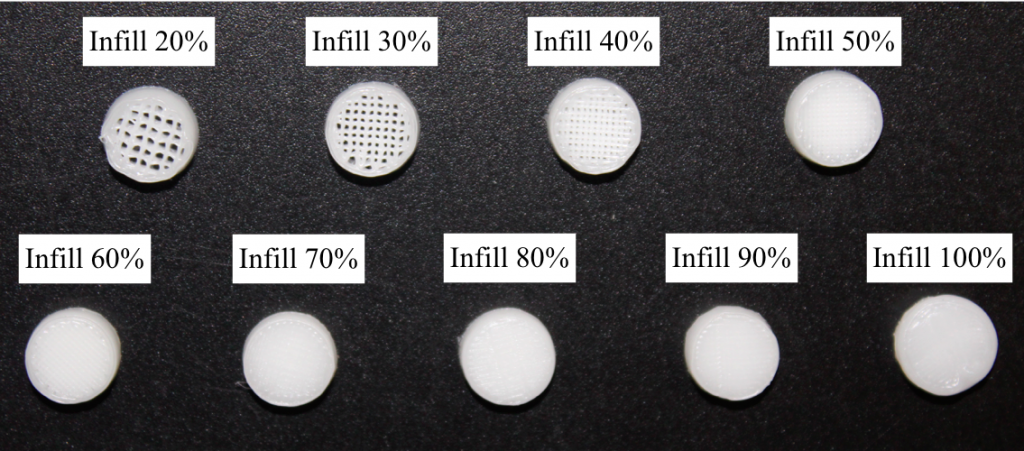
- We conducted tensile test on a set of patches. As mechanical characterization results we decided to consider the force-displacement curves for a more immediate comparison between synthetized and 3D printed patches.
Results
- Optimal printing parameters for PCL thermoplastic filament
- Optimal printing parameters for PLA-PCL bi-extruder printing
- Biological results
- Mechanical characterization
Collaborations
- Prof. Bice Conti, Department of Pharmaceutical Sciences, University of Pavia, Pavia, Italy
- Dr. Marco Benazzo, Director UOC Otorinolaringoiatria, Fondazione IRCCS Policlinico San Matteo, University of Pavia, Pavia, Italy
- Prof. Gino Volpato, Department of Clinical-Surgical, Diagnostic and Pedriatic Sciences, Unit of Thoracic Surgery, University of Pavia, Pavia, Italy